Accurate binding mode prediction:
CovDock is built upon a foundation of the time-tested Glide docking algorithm and Prime structure refinement methodology for accurate prediction of non-covalently docked poses. Glide quickly samples a large pool of initial poses for the pre-reactive species and Prime simultaneously optimizes the ligand pose and attachment residue to produce a sound physical chemistry. The resultant accuracy outperforms other docking programs in achieving lower RMS deviations from native co-crystallized structures.
Amazon.com Return Policy: You may return any new computer purchased from Amazon.com that is 'dead on arrival,' arrives in damaged condition, or is still in unopened boxes, for a full refund within 30 days of purchase. Amazon.com reserves the right to test 'dead on arrival' returns and impose a customer fee equal to 15 percent of the product sales price if the customer misrepresents the.
Complete workflow:
CovDock performs a series of automated steps based on a simple setup from the Maestro graphical interface or from the command line. First, CovDock docks the pre-reactive ligand to determine viable poses that bring the reactive group into close proximity with the reactive receptor residue. Then the covalent bond is formed for the top scoring complex structures, the covalently attached ligand is sampled, and the complexes are scored using all-atom molecular mechanics with the OPLS force field and VSGB2.0 implicit solvent model.
Intuitive graphical interface:
Schrödinger’s intuitive graphical user interface, Maestro, provides easy-to-use panels for straightforward set-up of experiments, easy visualization, and efficient analysis of CovDock results.
Cdockablepane
Citations and Acknowledgements
- Problem: macOS Dock is the command center for your Mac.With Apple Dock you can run applications, switch between apps, open documents and links. Unfortunately, Apple Dock lacks many useful features such as Window Management and Switching between Windows, appearance Customization, Hiding app icons. Solution: ActiveDock is a full replacement for Apple Launchpad and Dock with many new features.
- Tips for better search results. Ensure correct spelling and spacing - Examples: 'paper jam' Use product model name: - Examples: laserjet pro p1102, DeskJet 2130 For HP products a product number. Examples: LG534UA For Samsung Print products, enter the M/C.
- Dock, Cdock a4318 Artist. Edit Artist; Share. Marketplace 855 For Sale. Vinyl and CD Discography; 6 Releases 3 Singles & EPs 3 Miscellaneous 25 Appearances 2 Albums 4 Singles & EPs.
- Kelley dock levelers, HVLS fans, trailer restraints, dock seals and ergonomic lifts are solutions for a safe, efficient, energy-saving loading dock.
ö Zhu, K.; Borrelli, K.W.; Greenwood, J.R.; Day, T.; Abel, R.; Farid, R.S.; Harder, E., 'Docking covalent inhibitors: A parameter free approach to pose prediction and scoring,' J. Chem. Inf. Model., 2014, 54, 1932−1940
In this article, Principal Scientist Kai Zhu introduces CovDock, a powerful tool for accurately docking and scoring covalent binders.
Cdock Download
Introduction
Covalent drugs form a covalent bond with their target protein and derive their activity not only from non-covalent interactions, but also from the formation of a covalent bond. These drugs offer unique opportunities in the design of selective and potent binders. With the recent resurgence in covalent drug research, computational insight into covalent docking is becoming key to understanding how covalent inhibitors can be used to address selectivity and potency challenges. Here at Schrödinger we have developed a novel approach, CovDock1-2, for docking and scoring covalent inhibitors. This approach needs no parameter fitting for different covalent reaction types, is easy to set up, and is very accurate in pose prediction. We have also successfully adapted it to be suitable in the virtual screening of large databases with balanced speed and accuracy, and have validated the method using a variety of systems.
The procedure for CovDock’s pose prediction and scoring is built upon the well-validated Prime3 and Glide4 methodologies, and – in contrast to most other covalent docking programs – does not require additional parameter fitting for any specific chemical reaction types. As shown in Figure 1, CovDock first mutates the reactive residue to alanine, samples ligand conformations with ConfGen, and utilizes the rapid sampling provided by our docking program Glide to generate a large pool of initial poses for the pre-reactive species. Then based on geometric criteria, a subset of poses are selected and covalently linked to the receptor. The protein structure prediction and refinement program Prime is then used to simultaneously optimize the ligand pose and the attachment residue to produce a sound physical geometry. The resultant protein-ligand complex geometries are ranked based on an all-atom energy function VSGB2.0, which has been extensively validated in the context of protein structure prediction. The covalent bond parameters are taken from the OPLS force field such that any non-physical bond distances, angles, or torsions are appropriately penalized.
Figure 1:CovDock methodological workflow
To estimate the binding affinity, we developed a scoring procedure that utilizes information from both the Glide scores of the unbound pre-reactive species and the Glide score of the covalently bound final predicted binding mode. This scoring function does not model the bond formation energy directly, but it captures the fitness of the covalent bond implicitly. In particular, this scoring procedure assumes a promising covalent inhibitor must bind with high affinity prior to forming a covalent bond with the protein, and must not form especially energetically unfavorable contacts with the protein after the reaction has completed. We expect such an assumption to be reasonable when the intrinsic reactivity of the reactive warhead is largely unmodified across the congeneric series.
Cdock 2
In addition, we’ve developed CovDock-VS specifically tailored to address the throughput needs of structure-based virtual screening, balancing execution speed and accuracy. The CovDock-VS mode is an order of magnitude faster than the default mode and has been validated on several systems with the library size varying from 1500 to 5000 compounds. To the best of our knowledge, CovDock-VS is the first fully automated tool for efficient virtual screening of covalent inhibitors.
Pose Prediction
In a comparative study of CovDock with CovalentDock5, Autodock6, and GOLD7 applied to the same dataset (containing 76 complexes with 13 Michael addition and 63 acylation by β-lactam ring opening), the methods are assessed by calculating the RMSD of the top scoring pose, as well as the RMSD of the best pose among the top 10 scoring poses. As shown in Table 1, CovDock clearly outperforms the other methods with an average top scoring pose RMSD of 1.8 Å.1
Table 1: The pose prediction accuracy measured by average RMSD (Å) of CovDock and other programs on the CovalentDock data set.
A separate data set was used to test CovDock’s pose prediction accuracy against experimental data, the data set consisting of 38 complexes from the PDB (27 Michael addition, 11 nucleophilic addition). As shown in Table 2, in 76% of cases the top-scoring pose is correctly predicted within 2 Å of the crystal structure. Many of the failures can be understood in the context of the imperfect crystal structures or the dramatic conformational change the ligands undergo before and after the covalent reaction.1
Table 2:For the dataset consisting of the 38 studied complexes, pose prediction accuracy is reported for the top scoring pose as well as for the best pose within the 10 lowest energy poses. Accuracy metrics include the percentage of complexes where the predicted geometry is within 2 Å of experimentally-determined geometry and the average RMSD across all complexes.
SAR Study
The CovDock affinity score is a relative measurement of binding strength, with the assumption that the intrinsic reactivity of the inhibitor warhead is largely unmodified across the series. In this study, we sought to evaluate the performance of CovDock for ranking a congeneric series of compounds in order to establish if there is a correlation between calculated CovDock apparent binding affinity score and experimental activity. We studied 11 acrylamide inhibitors that undergo a Michael reaction with the cysteine residue Cys345 of the cSrc kinase binding pocket.
Cdock 2
Figure 2 shows the computed binding affinities plotted against the experimental binding affinities, with an observed R2 of 0.62. The docked poses of the strongest and weakest binders are shown at right, differing only at the other side of the molecule (i.e., near Asp404) close to the bottom of the binding pocket, where the strongest binding ligand (green) has a bromine atom (RTln(IC50app) = -9.7kcal/mol) and the weakest binding ligand (purple) has a tert-butyl group (RTln(IC50app) = -6.2kcal/mol). As can be seen, the bottom of the binding pocket can accommodate the bromine, but cannot accommodate the tert-butyl group; thus, the ligand with tert-butyl is pushed outside the pocket and this is reflected as a poor CovDock score.
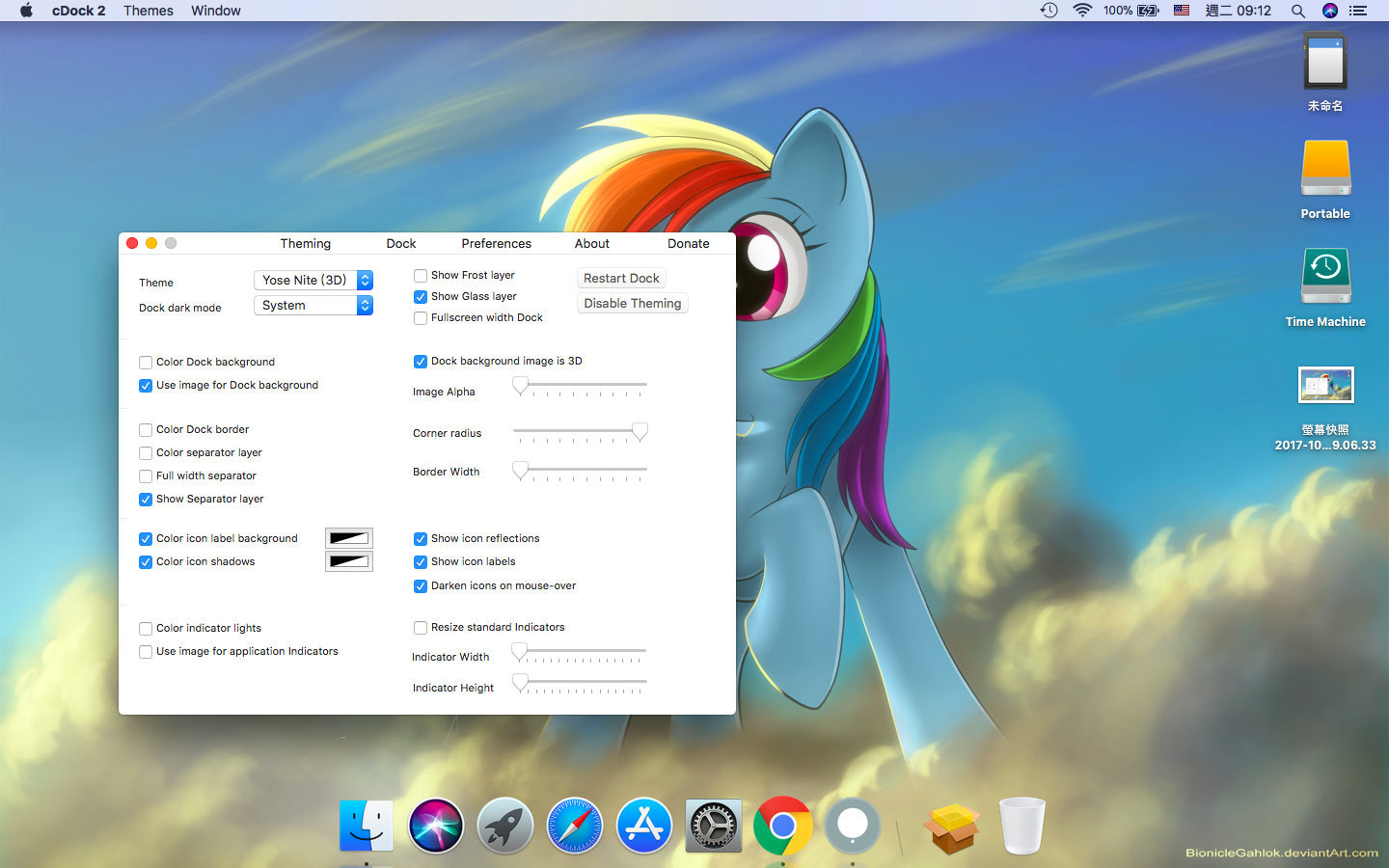
Figure 2: Apparent binding affinities of 11 acrylamide inhibitors of cSrc kinase are plotted at left against the experimentally-determined binding affinities. The docked poses of the strongest (green) and weakest (purple) binder are shown at right. The receptor is rendered as grey surface, except Cys345 and Asp404 which are shown as tubes.
Virtual Screening
The virtual screening mode CovDock-VS provides a fast and accurate way to differentiate actives from decoys (inactives) without significantly deteriorating the accuracy of the predicted poses for covalent protein-ligand complexes. For more details, please see Toledo Warshaviak et al.2
In this study, CovDock-VS was applied to four targets (HCV NS3 protease, Cathepsin K, EGFR, and XPO1) with known crystal structures and known covalent inhibitors. As shown in Table 3 and Figure 3, by using CovDock-VS in conjunction with interaction filters (in this case, H-bond constraints), we can retrieve 71%, 72%, and 77% of the known actives for Cathepsin K, HCV NS3 Protease, and EGFR within 5% of the decoy library, respectively (see EF 5% column in Table 3). Even when interaction filters could not be used, as for XPO1, we still retrieved 95% of the actives within 30% of the decoy library for XPO1, with 33% and 57% of the known compounds included in 1% and 5% of the decoys, respectively (see EF 1% and EF 5% columns in Table 3).
Table 3: The virtual screening results for four targets are reported. The performance is measured by Enrichment Factor (EF) with different cutoffs (1%, 5% and 10%).
Figure 3: Enrichment curves for A) Cathepsin K, B) HCV NS3 Protease, C) EGFR ensemble, and D) XPO1 ensemble.
Summary
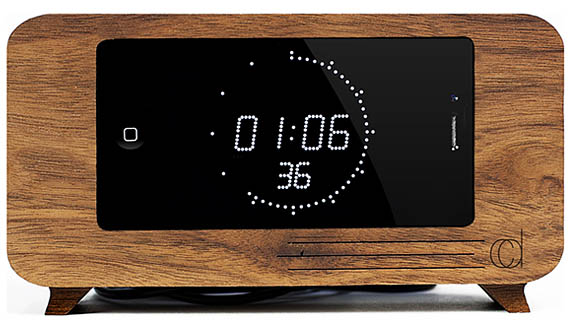
- CovDock outperforms other programs in the docking accuracy in head-to-head comparison and has been extensively validated with large data sets
- CovDock-VS (virtual screening mode) balances speed and accuracy and has been successfully used in the discovery of covalent bound ligands with various targets
- CovDock does not require parameter fitting for any specific chemical reaction types
- CovDock uses SMARTS to define the reaction atoms and can easily support any chemical reaction
- CovDock is easy to set up, fully automatic, and requires minimal manual preparation
References
- Zhu, K.; Borrelli, K.W.; Greenwood, J.; Day, T.; Abel, R.; Farid, R.; Harder, E. Docking Covalent Inhibitors: a Parameter Free Approach to Pose Prediction and Scoring. J. Chem. Inf. Model. 2014, in press.
- Toledo Warshaviak, D.; Golan, G.; Borrelli, K.W.; Zhu, K.; Kalid, O. A Structure-Based Virtual Screening Approach for Discovery of Covalently Bound Ligands. J. Chem. Inf. Model, 2014, in press.
- Schrödinger Release 2013-2: Prime, version 3.3, Schrödinger, LLC, New York, NY, 2014.
- Small-Molecule Drug Discovery Suite 2013-2: Glide, version 6.0, Schrödinger, LLC, New York, NY, 2014.
- Ouyang, X.; Zhou, S.; Su, C. T. T.; Ge, Z.; Li, R.; Kwoh, C. K. CovalentDock: Automated Covalent Docking with Parameterized Covalent Linkage Energy Estimation and Molecular Geometry Constraints. J. Comput. Chem. 2013, 34, 326–336.
- Morris, G. M.; Huey, R.; Lindstrom, W.; Sanner, M. F.; Belew, R. K.; Goodsell, D. S.; Olson, A. J. Software News and Updates AutoDock4 and AutoDockTools4 : Automated Docking with Selective Receptor Flexibility. 2009, 30, 2785-2791.
- Jones, G.; Willett, P.; Glen, R. C.; Leach, A. R.; Taylor, R. Development and Validation of a Genetic Algorithm for Flexible Docking. J. Mol. Biol. 1997, 267, 727–748.
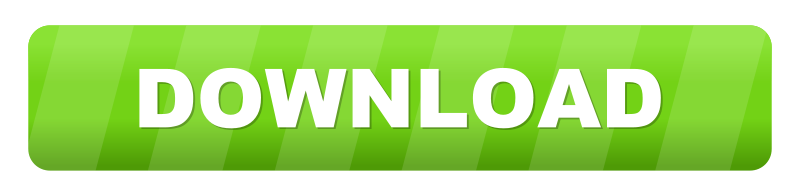